The prospects of additive manufacturing in nuclear power plants are promising, with the potential to revolutionise the industry in numerous ways. Additive manufacturing offers the ability to produce complex and customised components with enhanced performance and durability, addressing challenges related to traditional manufacturing methods. This technology enables rapid prototyping, on-demand production and localised manufacturing, reducing lead times, costs, and the need for extensive inventories of spare parts. Furthermore, additive manufacturing facilitates the creation of intricate geometries that are difficult to produce using conventional methods, leading to improved efficiency and performance of critical components.
Additionally, additive manufacturing plays a crucial role in the development of advanced materials for nuclear applications, enhancing safety and resilience. As the nuclear industry continues to embrace digitalisation and advanced technologies, additive manufacturing is set to drive innovation, improve operational flexibility, and enhance the overall sustainability and competitiveness of nuclear power generation. Embracing additive manufacturing technologies will be instrumental in unlocking the full potential of this innovative approach, ultimately reshaping the future of nuclear power plants.
Example Problem
The article discusses how KKL (Kernkraftwerk Leibstadt) nuclear power plant has used additive manufacturing to create a small-bore piping bracket with the goal of achieving a high natural frequency. It provides insights into the design and manufacturing process, showcasing the potential of additive manufacturing in addressing challenges related to traditional manufacturing methods. This innovative approach allows to produce complex, customised components with enhanced performance and durability.
The following image depicts the initial situation, showcasing a small-bore pipe attached to a large-bore pipe, with a heavy drain valve attached. The original design is deemed safe for the intended 40-year lifespan. However, in preparation for continued operation beyond this period, a bracket is set to be installed to ensure the structure remains robust and fit for further decades to come. This proactive measure demonstrates a commitment to long-term safety and reliability.
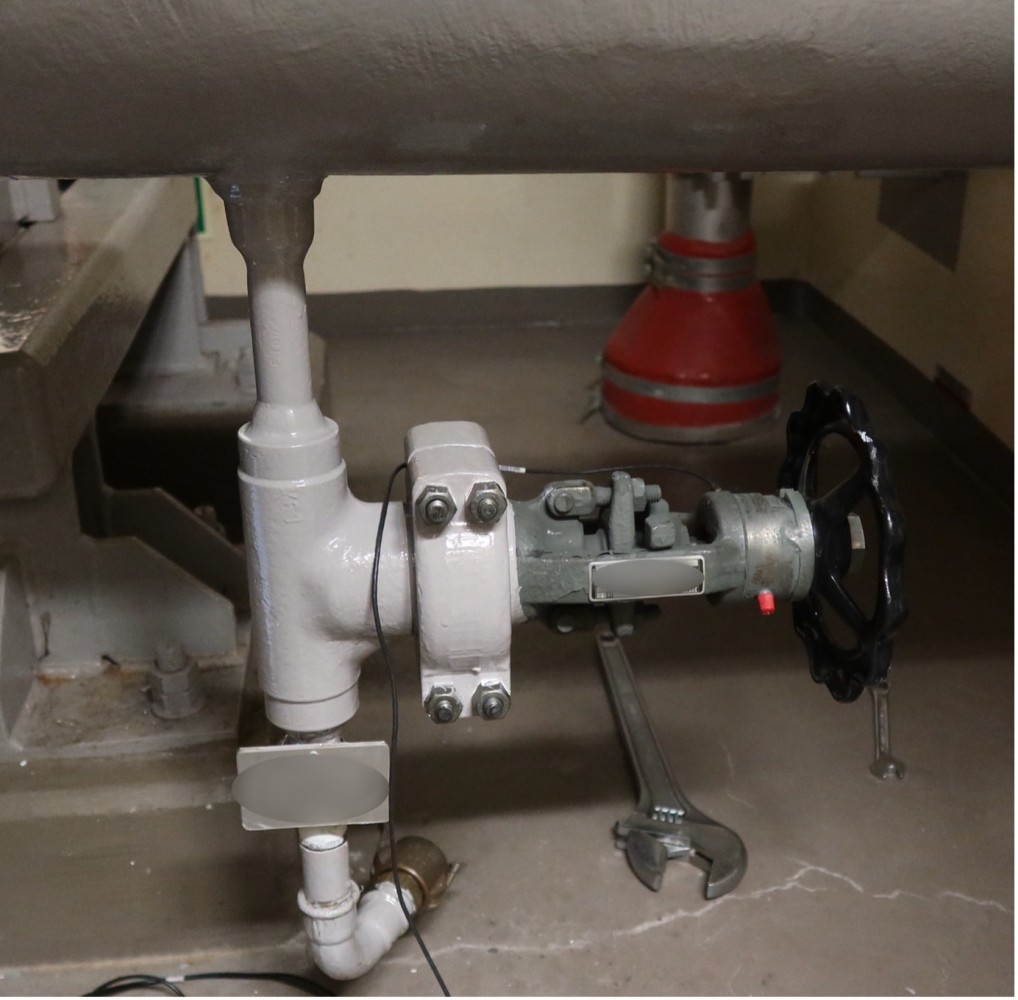
Design Volumes
Based on engineering reasoning, it was determined that the new bracket would consist of two separate pieces. A meticulous definition of the design volumes was crucial in this decision-making process. The key aspects considered for the design volumes included ensuring the build envelope was compatible with the 3D printing build volume, adhering to topological constraints, addressing various mechanical considerations and maintaining an optimal geometrical appearance. These factors were carefully evaluated to ensure that the design volumes would effectively accommodate the production and functionality requirements of the bracket.
The following image depicts the two distinct design volumes. Notably, the clamps for the main line pipe for the main bracket were incorporated at a later stage. This indicates an iterative design process, where additional topological and geometrical constraints were imposed to refine the bracket’s structure.
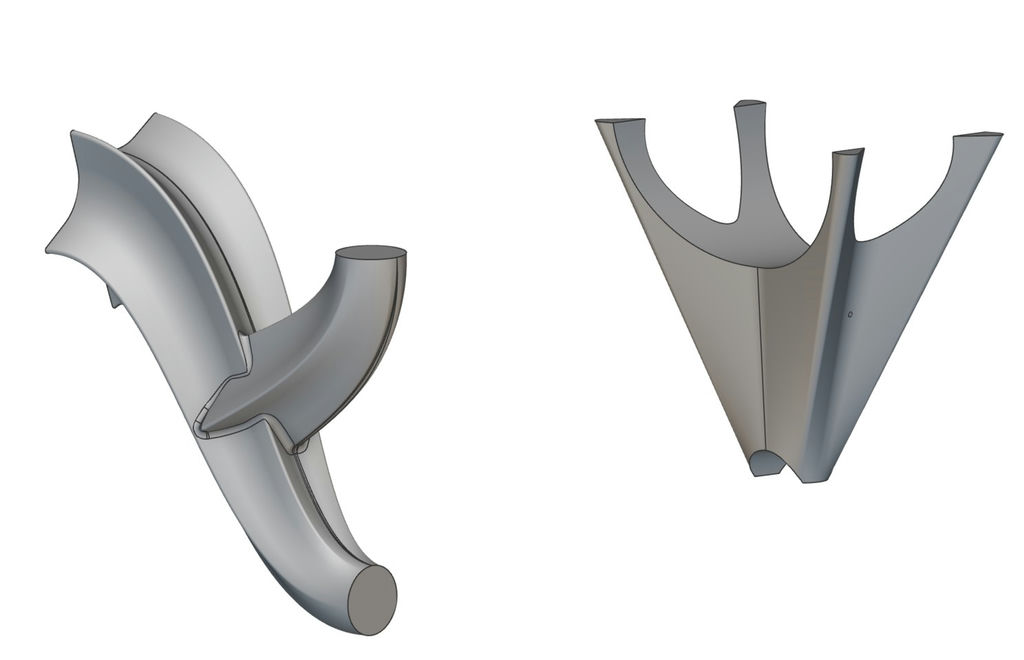
Design Results
Topology optimisation is a design process that utilises advanced software such as nTopology to iteratively determine the optimal material layout within the previously described design volumes, based on specified performance targets and constraints. The software enables engineers to define the desired structural or functional goals, such as minimizing material usage, reducing weight, or improving mechanical performance. By leveraging mathematical algorithms, topology optimisation systematically refines the design by redistributing material, resulting in structures that are both efficient and high-performing.
The inherent connection between additive manufacturing and topology optimisation lies in their ability to work in tandem to produce innovative and optimised components. Additive manufacturing allows for the realisation of the complex geometries generated through topology optimisation, while topology optimisation provides the design freedom and efficiency required to fully leverage the capabilities of additive manufacturing.
The next two images illustrate the raw and smoothened outcomes of the topology optimisation process. The raw image displays the initial output of the optimisation algorithm, showcasing the material distribution within the defined design volumes. In contrast, the smoothened image demonstrates the refined and optimised result, where the material layout has been slightly adjusted to enhance geometrical smoothness and thereby facilitate structural integrity.
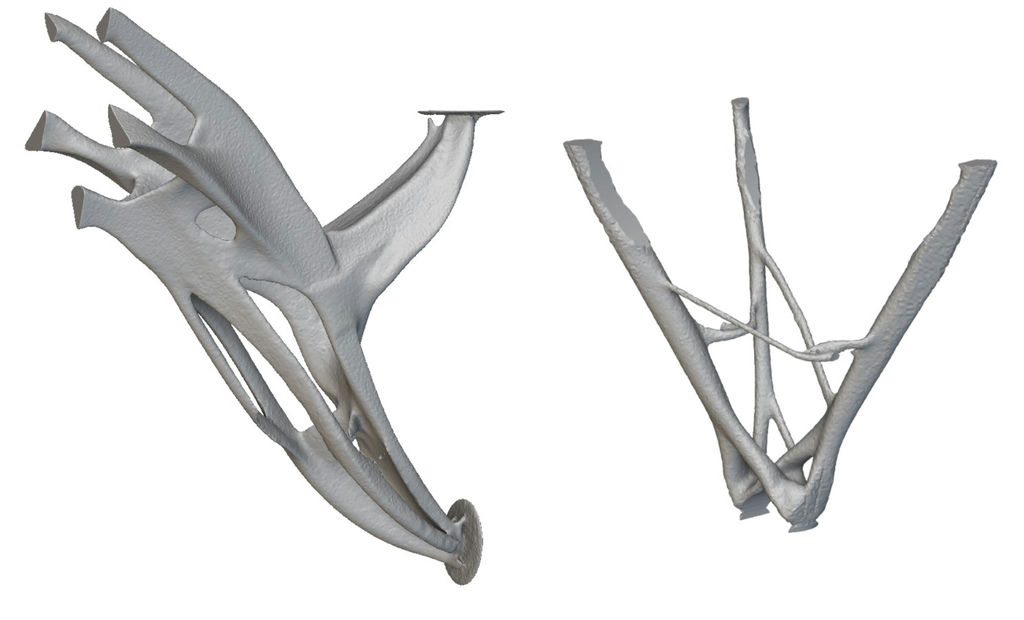
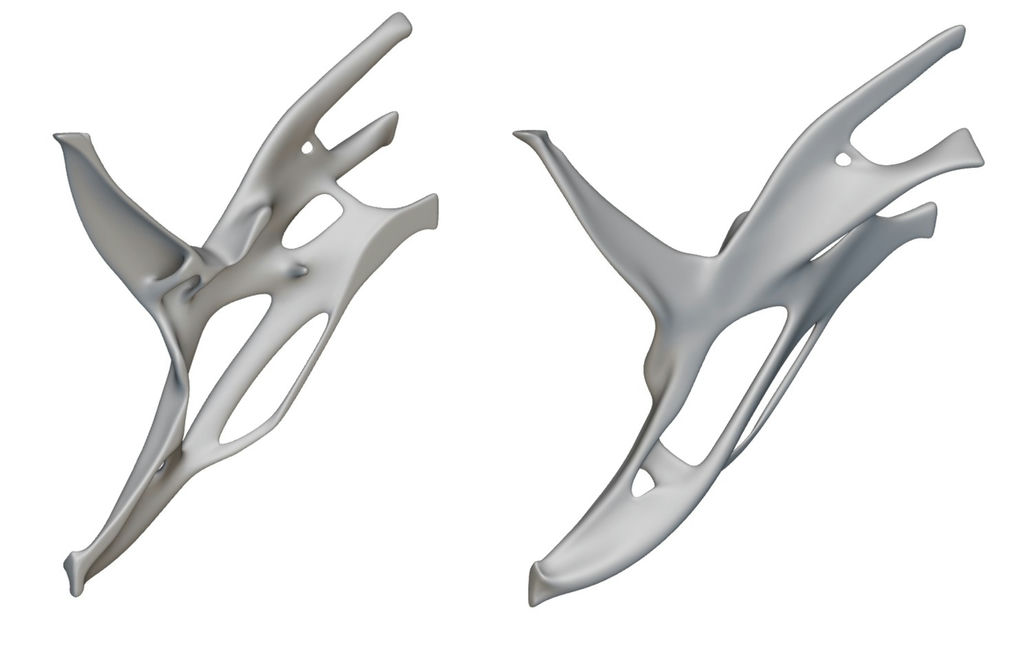
Final Design
The smoothened output geometry of the topology optimisation process was subjected to a design verification through stress and modal analysis. Stress analysis involved evaluating the structural integrity of the optimised design by assessing how static and dynamic forces and moments affected its mechanical behaviour. This analysis ensured that the design could withstand operational conditions without experiencing excessive deformation or failure.
Modal analysis focused on understanding the natural frequencies and mode shapes of the optimized structure. This analysis aided in identifying potential resonance issues that could lead to excessive vibrations or structural failure. By conducting modal analysis, engineers were able to ensure the component’s reliability and performance under the occurring loads.
The next image showcases the final design as CAD-model.
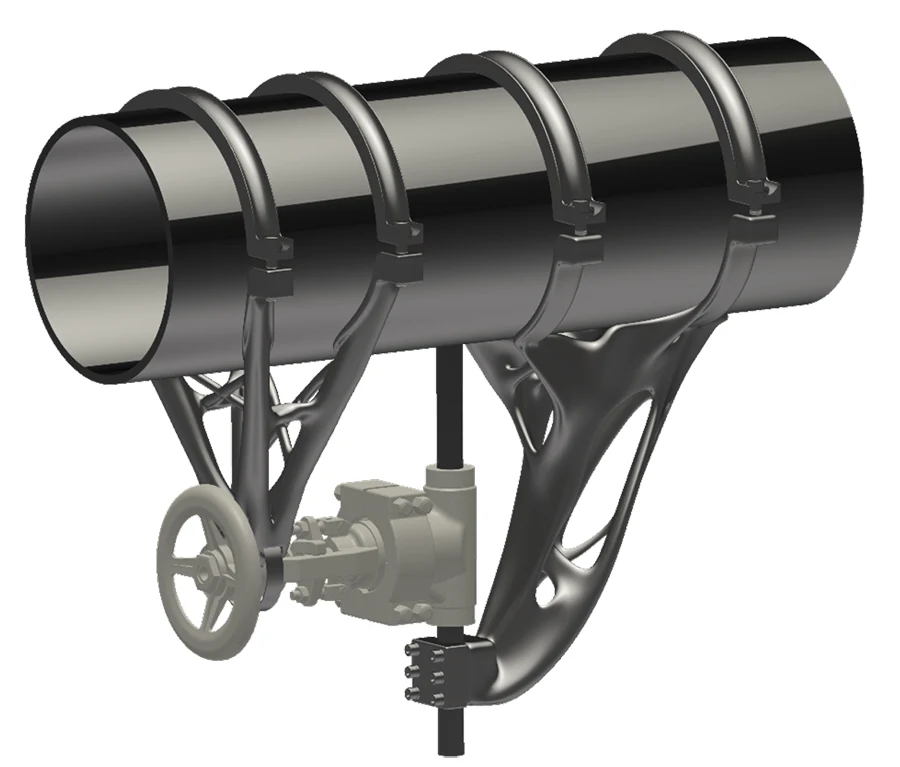
Subsequently, the identification of critical dimensions in the final design involves pinpointing features that are essential for its functionality, performance and manufacturability. Once these critical dimensions are established, the technical drawings are derived. The next image shows the assembly drawing exemplarily.
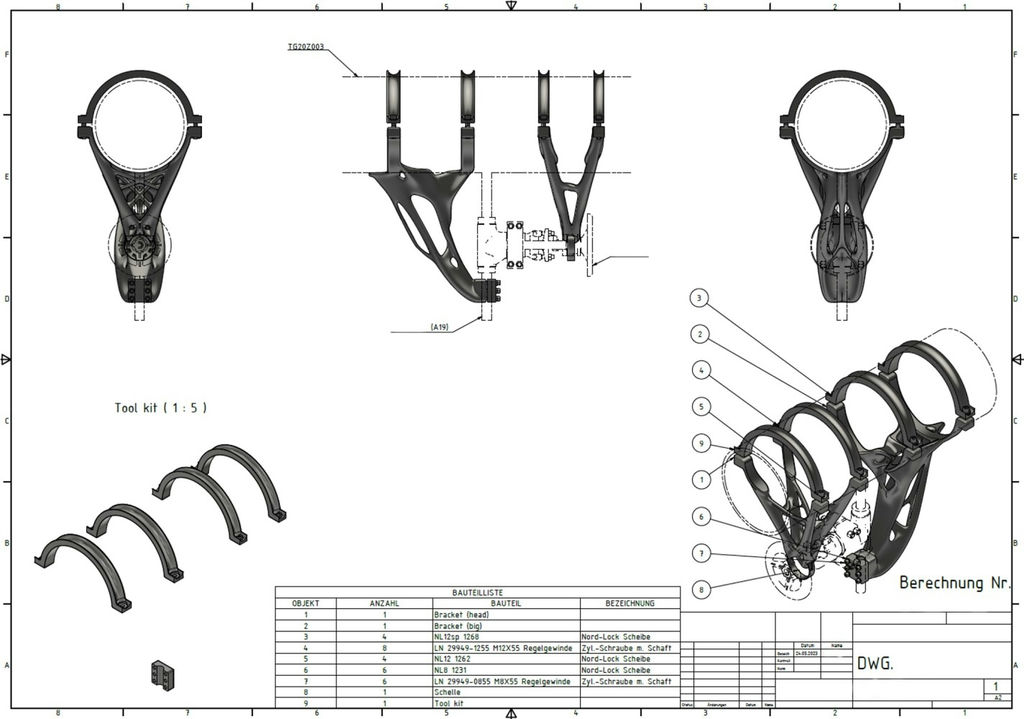
Manufacturing – 3D-Printing
The final design was manufactured using laser powder bed fusion (LPBF), a cutting-edge additive manufacturing process. LPBF involves the use of a high-powered laser to selectively melt and fuse powdered material, layer by layer, to create intricate and complex components. The next image provides an impression of the printing process in progress, showcasing the precision and detail involved in this additive manufacturing technique.
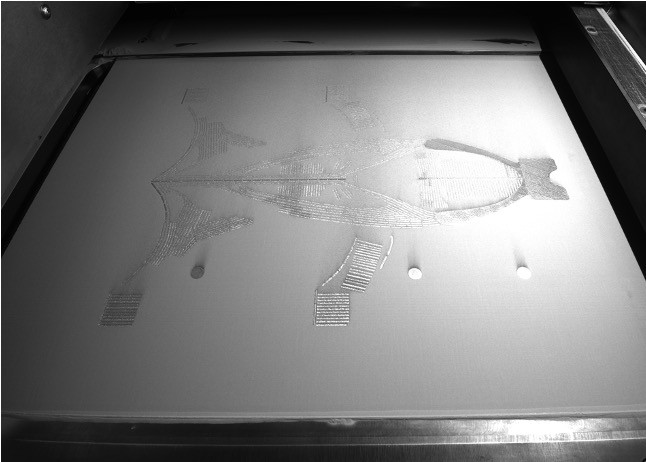
In LPBF additive manufacturing, support structures play a crucial role in ensuring the successful fabrication of complex and intricate components. These support structures are temporary elements that are added to the design to provide stability and prevent deformation during the printing process.
Support structures are typically generated automatically by the additive manufacturing software based on the design’s geometry and orientation. They are strategically placed to anchor overhanging features, bridges and other areas where the molten material may sag or collapse without additional support. This is particularly important in LPBF, where the laser selectively melts and fuses powdered material layer by layer, making it essential to maintain stability during the process. The next image shows the finished parts with all support structures still attached, highlighting the meticulous nature of the additive manufacturing process and the need for post-processing.
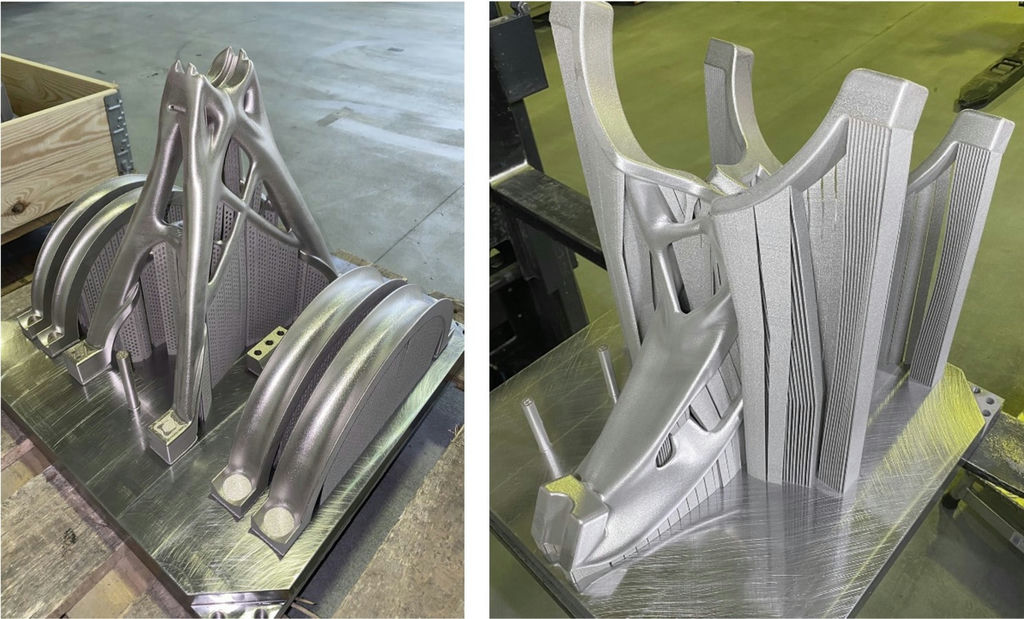
The next image shows the parts cut from the base plate with roughly removed support structures.
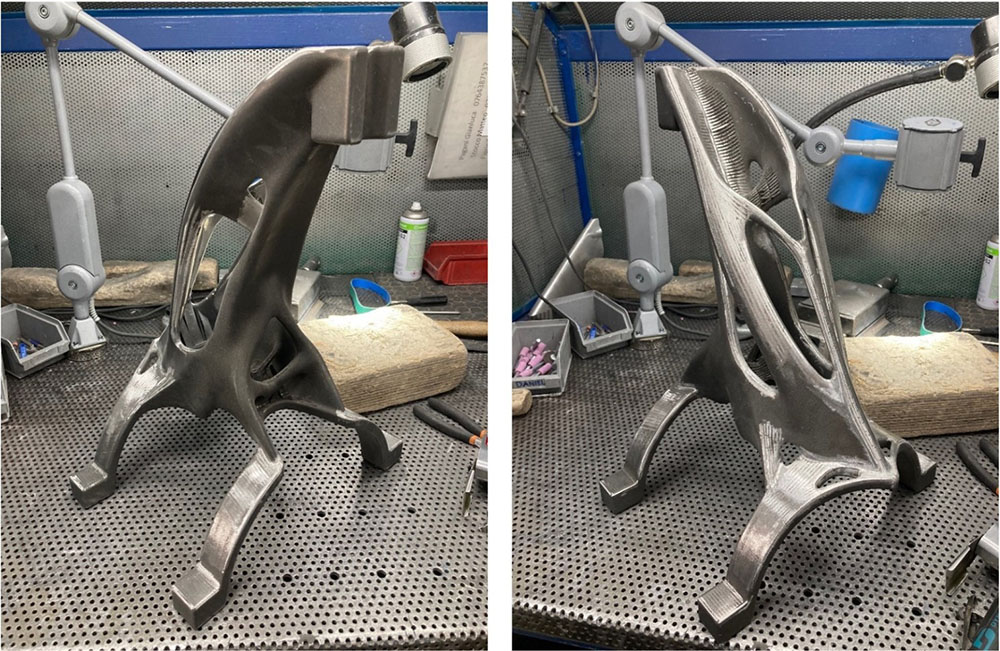
After the rough removal of the support structures, the parts still require post-processing to remove any surface irregularities. This post-processing step is essential for achieving a smooth surface finish, as it eliminates roughness and imperfections that may compromise the part’s functionality and aesthetics.
Furthermore, the reduction of surface roughness through post-processing is critical for enhancing the fatigue life of the parts. Surface irregularities can act as stress concentrators, leading to premature failure under cyclic loading. By carefully removing these imperfections, the fatigue life of the components can be significantly improved, ensuring their long-term durability and reliability.
Advanced post-processing techniques such as machining, grinding and chemical treatments are often employed to achieve the desired surface finish and reduce irregularities. Additionally, surface smoothing and polishing processes can be utilised to further enhance the part’s fatigue resistance and overall performance.
The next image shows the main bracket after surface finishing is completed.
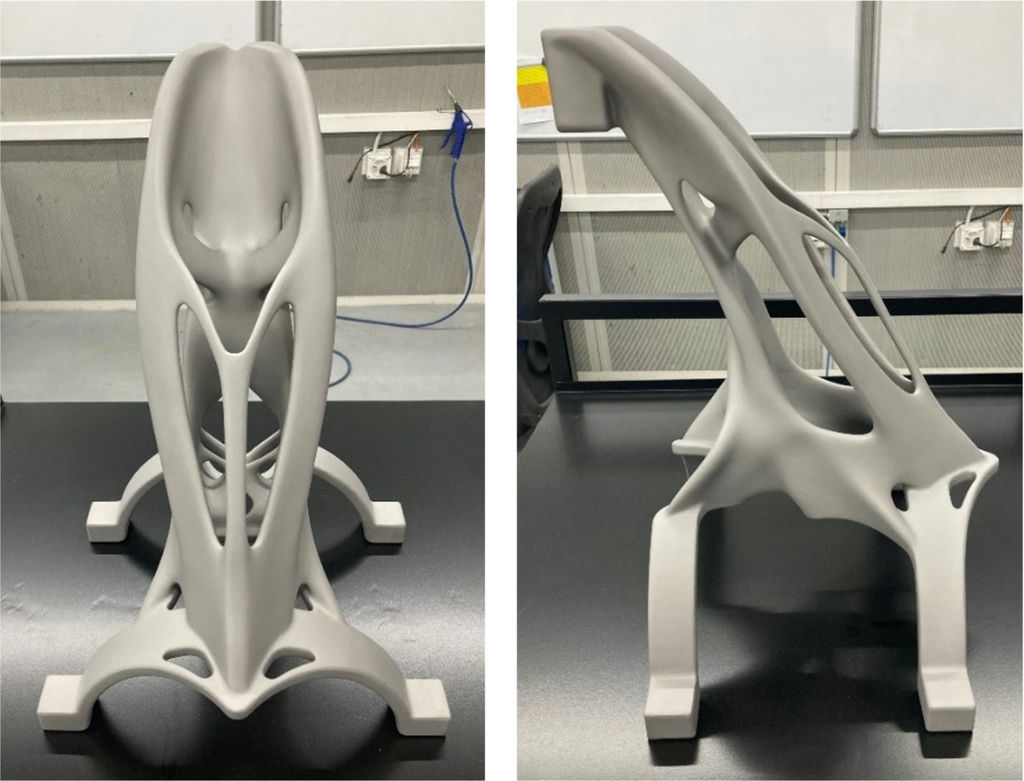
Manufacturing – Machining
Further, during post-processing, machining plays a vital role in achieving the necessary critical dimensions and surface finish in LPBF-manufactured parts. Machining processes such as milling, turning and grinding are used to ensure that additively manufactured parts meet critical tolerances. The following two image collections show the parts after machining of the critical surfaces is completed.
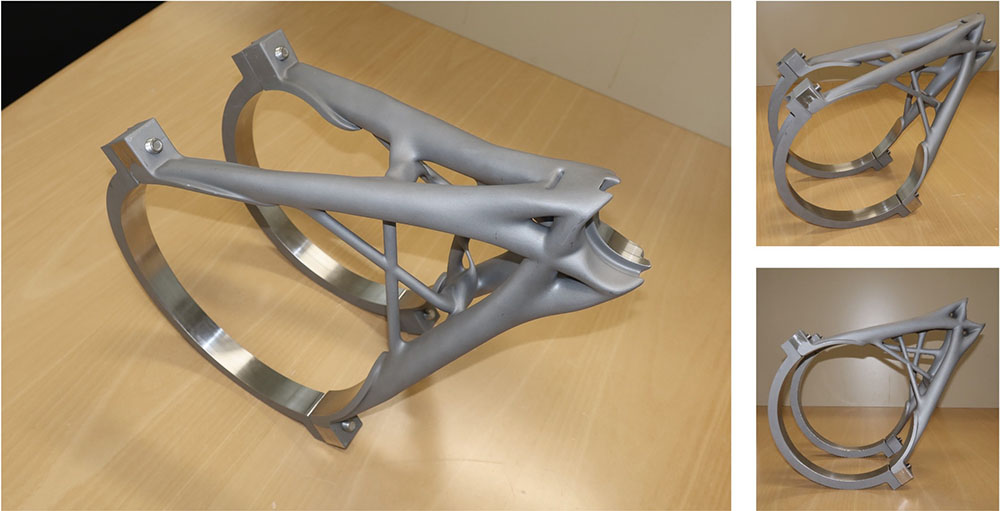
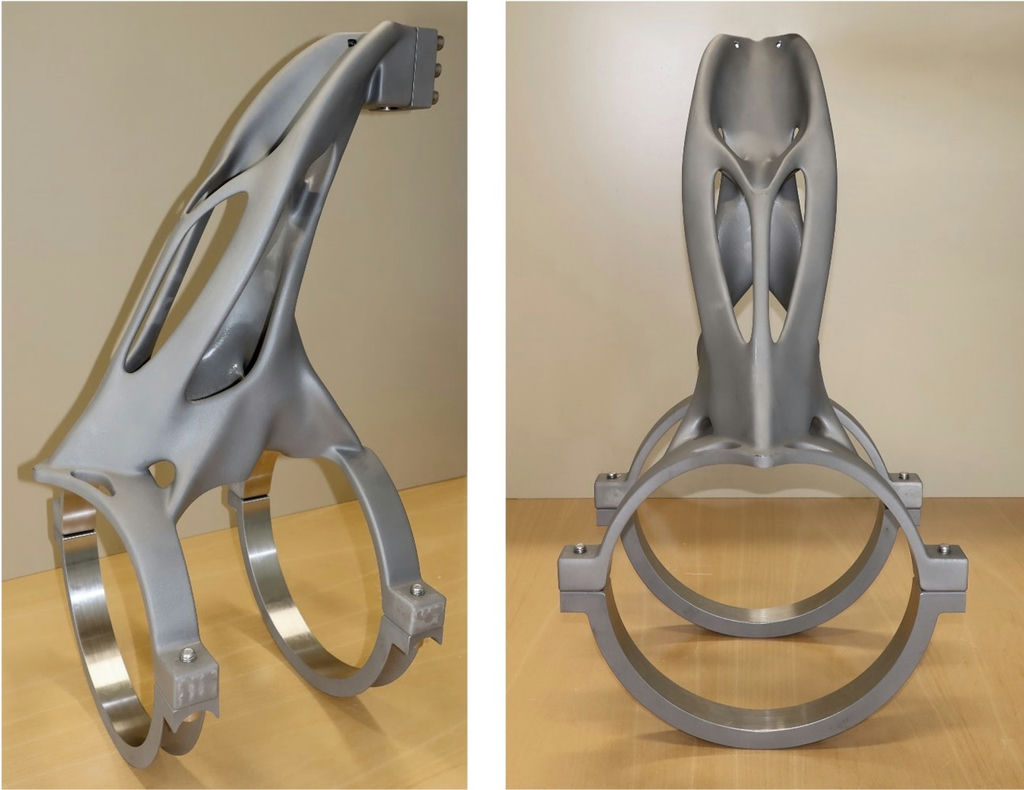
Installation
After machining and undergone final inspections, the parts were deemed to be installed. The meticulous machining process ensured the parts met precise dimensions and tolerance requirements, facilitating a proper fit during installation.
Additionally, the appropriate torque for bolted connections was carefully applied during the installation process. By following the recommended torque values, the integrity and function of the connections was set forth.
Following installation, a comprehensive validation of functionality was conducted to ensure that the parts performed as intended within the larger system. This involved testing the components under various operating conditions to verify their functionality, performance and durability.
The next images provide the overview shot of the assembly including surrounding components and detailed views from various angles.
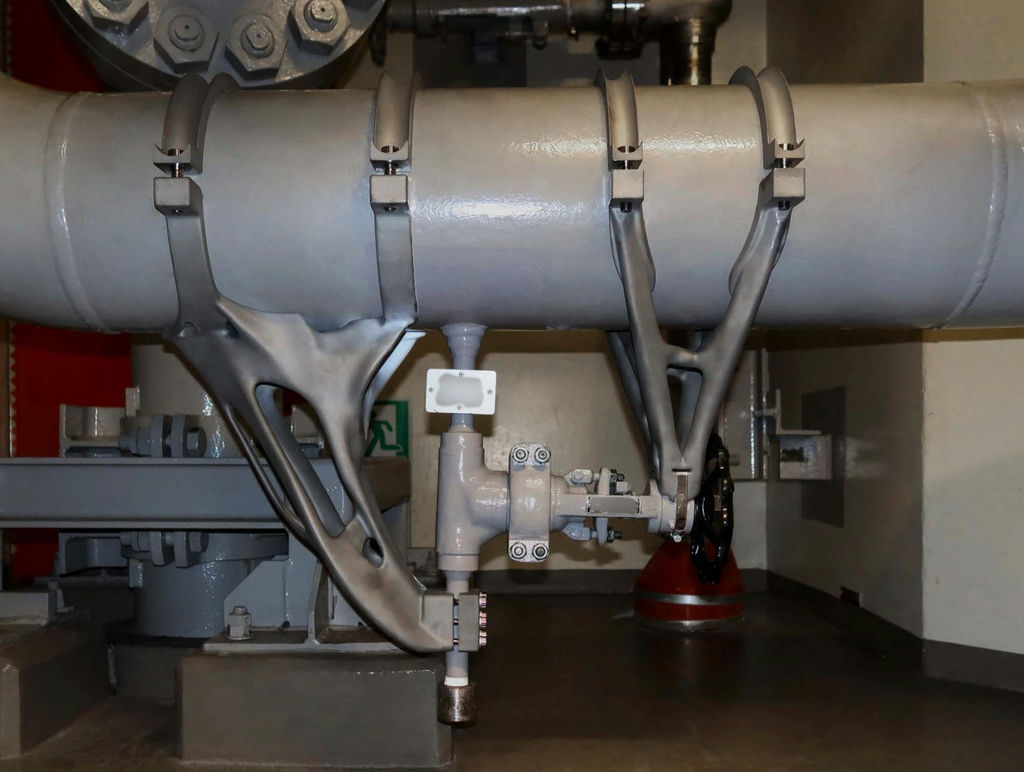
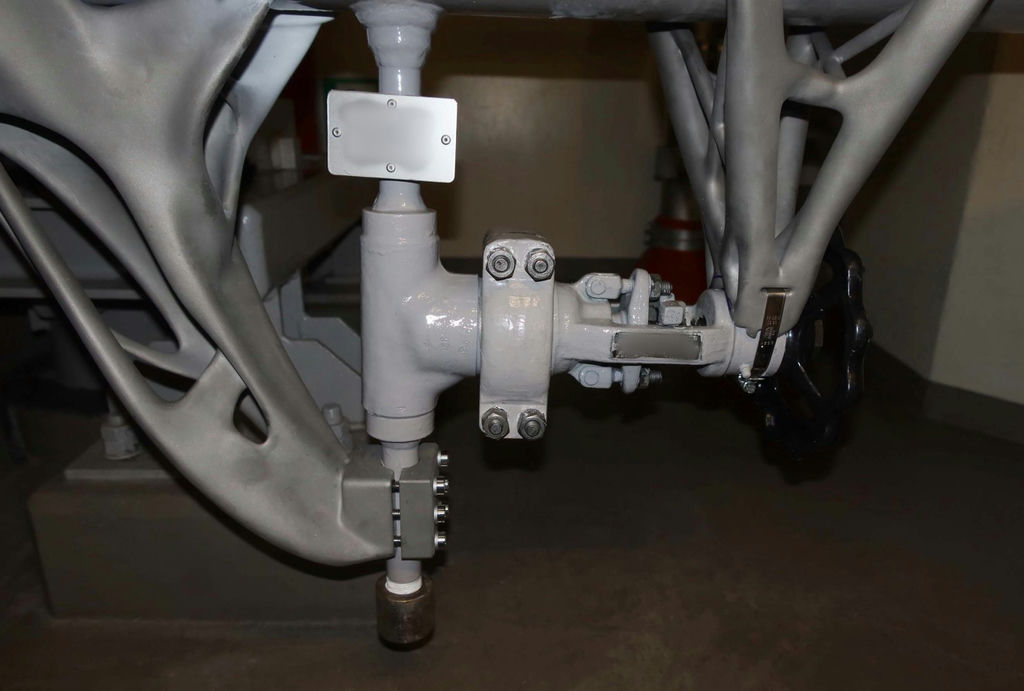
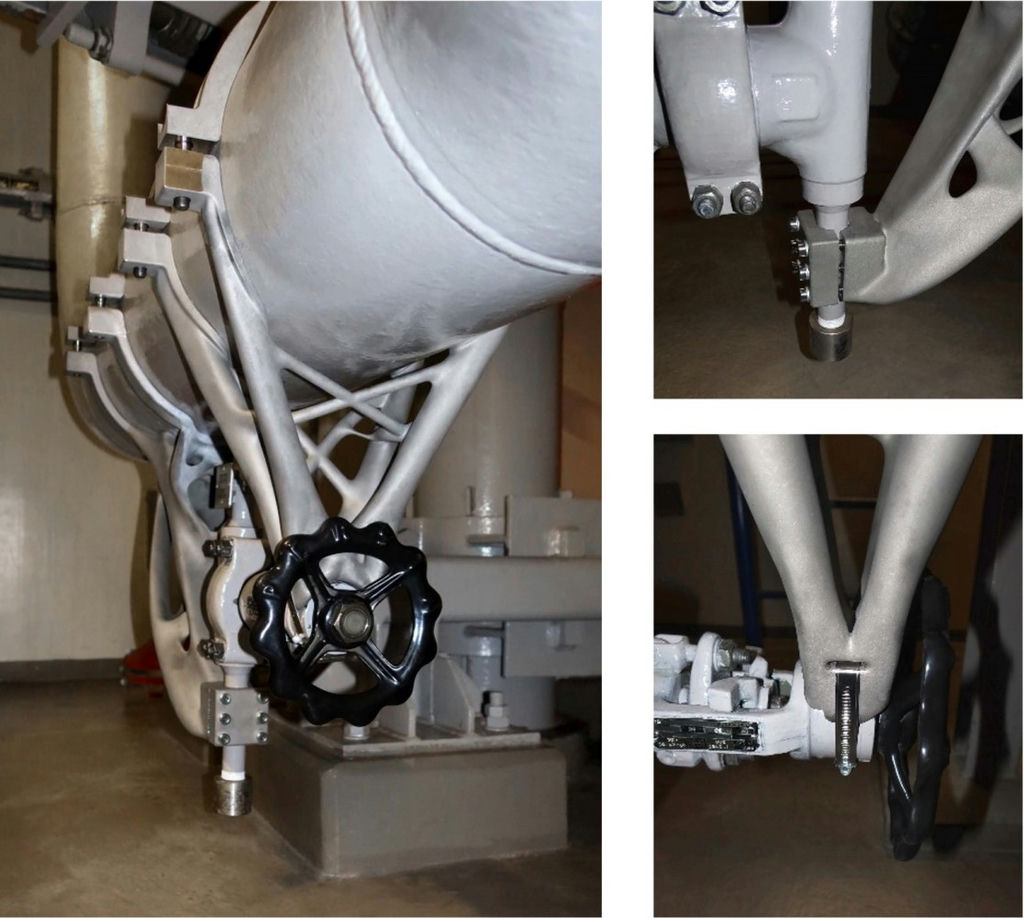
Summary
The article discusses the potential of additive manufacturing in nuclear power plants exemplarily, highlighting its ability to produce complex and customised components with enhanced performance and durability. It also details the design process of a small-bore piping bracket at the KKL nuclear power plant, emphasising the use of topology optimisation and laser powder bed fusion. The post-processing steps, including the removal of support structures and machining, are crucial for achieving the necessary critical dimensions and surface finish in LPBF-manufactured parts. After installation, a comprehensive validation of functionality was conducted to ensure the parts perform as intended within the larger system. Overall, the article emphasises the precision and attention to detail involved in the manufacturing and installation processes, ensuring the seamless integration and functionality of the components in the nuclear power plant.
To the audience by Author Benjamin Regener
The matter presented is highly complex and covers a wide range of topics. If there is a specific aspect that piques your interest and you would like more information on, please feel free to let me know. I’d be happy to delve deeper into any particular topic and provide additional details based on your feedback.
“This article originally appeared in as an LinkedIn article shared by Benjamin Regener, it was republished after the permission from the author”
Subscribe to AM Chronicle Newsletter to stay connected: https://bit.ly/3fBZ1mP
Follow us on LinkedIn: https://bit.ly/3IjhrFq
Visit for more interesting content on additive manufacturing: https://amchronicle.com